This research project is being conducted by Lisa Kearney, a PhD candidate from the School of Earth & Atmospheric Sciences at QUT, working with Dr. Luke Nothdurft as supervisor and Dr. Crystal Cooper, Microscopy Lab Co-Ordinator at their Central Analytical Research Facility (CARF). The project investigates the formation of limestone rock through natural cementation in modern coral reefs. These are the processes responsible for reef formation and stabilisation. This research uses samples of marine carbonate material collected from Heron Reef, within the southern Great Barrier Reef, Australia. The project commenced in 2017, with the TESCAN TIMA arriving in 2020.
The TESCAN TIMA is an automated mineralogy solution, typically used by mine sites and geoscientists. In this article, we explore a non-conventional application that has wide-ranging importance across the world’s corals reefs.


calm day, Heron Reef, GBR. (Bottom) QUT research student Patrick Cooley collecting reef core on the reef, northern leeward reef edge at Heron Reef, GBR, in 2013. This core was recovered as part of a transect of cores collected at low tide using a hand-operated rotary drill as close to edge of the reef as possible. The wave break zone in the image is where the reef profile drops to 20 to 30m water depth. Photos taken by Dr Luke Nothdurft.
Why is it important to know about the Holocene marine carbonate reef rock and their mineral content?
Coral reefs are the most biodiverse ecosystems of the ocean. The Great Barrier Reef is the largest reef complex, spanning 2,300 km along the northeastern coast of Australia. It is composed of many individual reefs, which in turn are home to a vast array of organisms. These individual reefs exhibit a wide and complex range of reef morphologies from the macroscale down to the microscale making them ideal to compare to fossil and modern reefs.
Corals themselves are made up of colonies of individual polyps, which are made up of soft parts that don’t preserve very well in the fossil record, but their skeletons (see figures 2 and 3), the hard parts, do. As each individual polyp grows, it leaves behind a layer of material known as calcium carbonate. This calcium carbonate is what limestone is made of and, over time and under favourable conditions, corals produce thick limestone build-ups, which in turn act as substrate for new organisms to grow on.

Corals alone cannot make a coral reef. Marine geologists describe coral reefs as being a rigid, wave resistant skeletal structure whose framework is comprised of hard corals and many other components, including algae and other organisms. The interlocking and gluing of the many components is what stabilises the reef. Natural minerals that precipitate into voids and spaces provide the crucial glue that cements the skeletons, shells and sand together. This process of turning reef communities into a rigid limestone structure occurs in both modern and ancient reefs. The chemistry of fossilised reef communities (skeletal remains of plants, animals and natural cements) reflects the conditions of the ambient seawater in which they grew, and can be used to trace valuable information about the past such as water chemistry and climate information.
Natural cementation in reefs has structural, chemical, and economic significance. Cements contribute to the rigidity of reef build-ups, the maintenance of the positive relief above surrounding seafloor sediments and provide the high preservation potential of the reef structure in the fossil record. Yet we do not fully understand the processes around cementation and the overall lithification of reefs.
What is coral reef cementation and stabilisation?
Modern marine reef carbonates are typically composed of calcium carbonates, CaCO3; the primary phases being Aragonite, and High and Low Magnesium Calcite, but other mineral phases may be present (e.g., Dolomite, Brucite, Silica). These carbonate cements are typically present as crusts (~1 cm thick) or as pore lining (nano-micro) crystals within or on the skeleton components and grains. When these minerals are exposed to environmental conditions (e.g., seawater, pore-water, air) they are altered to the next stable phase (i.e., original skeletal aragonite into calcite). These diagenetic changes can occur during the formation of the respective reef component and continue post-mortem through fossilisation of skeletal parts.
Cement textures produced through diagenesis in differing environments can be used to determine where they formed. These various textures are typically segregated and can occur both intra- and inter-skeletally (i.e. outside or inside the skeleton within the rock, respectively). Compared to the million year time scales of typical geological rock formation, the complex range of textures observed in reef environments can form in days to weeks following deposition, especially when aided by biological processes.
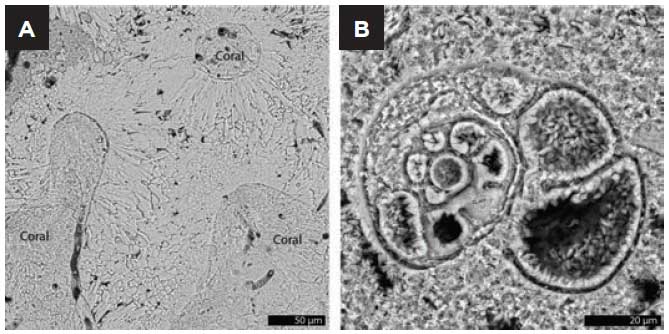
What are the aims of your research project?
The overall project aims to gain an improved understanding of the processes of diagenesis in a modern marine environment by looking into the past. We want to know what happens from the earliest deposition of reef materials, through to the formation of the final cemented reefrock (limestone). The current study focuses on answering what is gluing the reef together, or literally, what is cementing the reef. Samples have been selected showing early stages of lithification. These sections contain grains and/or fossil skeletons of the reef community that are loosely held in place within reef cavities, see figure 2A. These sections provide a unique opportunity to investigate early diagenetic products.
Mineralogical and chemical changes (diagenesis) during the fossilisation process significantly disturb the chemical information retained by the skeleton, as original skeletons are altered. Diagenetic alteration to reefal components, especially in coral skeletons, affects the reliability of the geochemical data that is used for palaeoenvironmental and isotopic dating. For example, paleoenvironmental reconstructions can be made by looking at elevated strontium (Sr) concentrations in secondary aragonite or alterations in magnesium (Mg) content in calcite, which reflect cool or warm temperature anomalies, respectively. A greater understanding of the diagenetic processes occurring in these environments, and in turn a vetting protocol, is needed to minimise these geochemical issues.
Rare earth element (REE) proxies and other geochemical information can be retained by microbialites and, in some cases, fossilised skeletons can retain geochemical information through diagenesis. The data generated from the TIMA can be used to target analytical points for further REE and trace element analysis using methods like laser ablation-inductively coupled plasma-mass spectrometry (LA-ICP-MS).
Is your research relevant to other coral reefs elsewhere in the world?
Yes. This research is relevant to all tropical reef carbonates, especially reefs with similar geomorphology (platform reefs). The technique can be applied to any reef material and is currently being used to investigate ancient reef carbonates.
Coral and other reef builders are a biological material. How has the TIMA, designed for mineralogical materials been suitable tool for your research?
Corals, and most carbonate materials, are formed through biological processes, but the end material/part that is preserved is the fossilised skeleton. This skeleton is composed of a metastable calcium carbonate mineral, aragonite, orthorhombic CaCO3. Skeletal aragonite contains minor amounts of strontium (Sr), as it is a biologically relevant mineral that can be used to chemically differentiate it from calcite, rhombohedral CaCO3. Subtle variations in strontium and magnesium content can be used to distinguish carbonate mineral phases (aragonite, calcite, LMC, HMC, dolomite).
Why is it important to be able to look at corals on a micro-level?
Scanning electron microscopy is a non-destructive method that provides microtextural and elemental information. Texture can be used as a distinguishing feature for many cements and skeletal parts of the samples.
The research question currently being addressed looks at the cements between grains in the samples, much of which is micritic. Being able to view the samples at various length scales is key for their accurate identification.
If you had not had access to the TIMA, what would you have used?
Previously, I was using the TESCAN MIRA3 system as it has low vacuum large field of view imaging capabilities. The previous workflow involved a combination of acquiring large area back scattered electron (BSE) maps of the samples and with manual point counting. The BSE maps were used to select regions of interest for further image acquisition and energy dispersive spectroscopy (EDS). These were used for the identification of minerals/materials during point counting.
Compared to using MIRA3, how has the TIMA been beneficial to your research?
The TIMA allows for relatively non-destructive, detailed mapping of carbonate minerals at high-resolution. The multiple EDS detectors easily allows for subtle phase distinctions within the carbonate mineral groups. Collection of detailed microtextural BSE, elemental, and phase (mineralogy) information from the TIMA streamlines understanding the carbonate quantification process (figure 4).
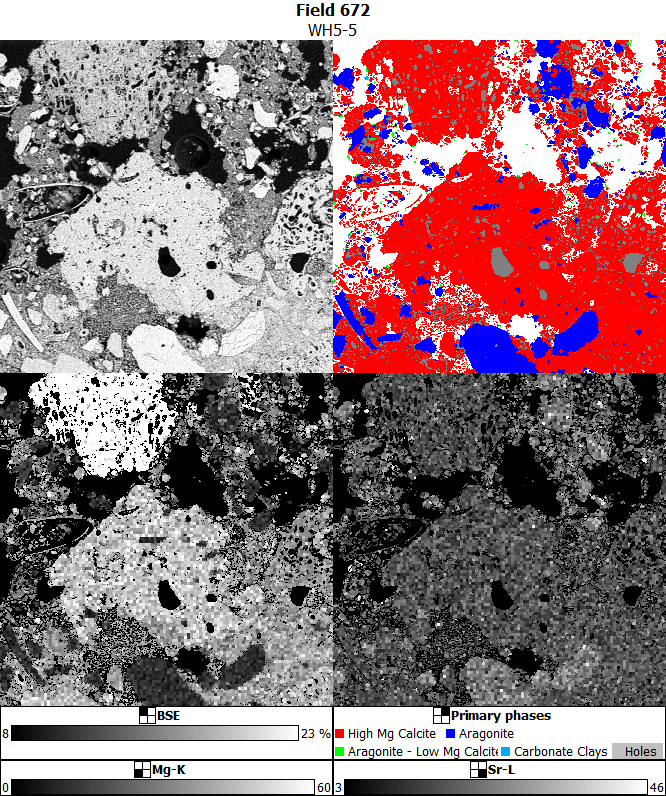
The automation of data collection is a major benefit. Numerous datasets can be acquired for multiple samples in a time-effective manner. The TIMA possesses multiple EDS detectors that allow rapid and reliable collection of high quality spectral data while minimising sample damage. The unique software capabilities allow for the processing of spectra, where a mineral phase library can be built upon sample spectra and reference materials or online standards.
Authors
Lisa Kearney1, Crystal Cooper1, Cameron Chai2, Kamran Khajehpour2 and Luke Nothdurft1
- Queensland University of Technology
- AXT PTY LTD
This article was published in the September 2021 edition of the Australian Microscopy and Microanalysis Society Newsletter.